Introductory essay
Written by the educators who created The Deep Ocean, a brief look at the key facts, tough questions and big ideas in their field. Begin this TED Study with a fascinating read that gives context and clarity to the material.
How inappropriate to call this planet Earth when it is quite clearly Ocean.Arthur C. Clarke
Planet Ocean
In the late 1960s, the Apollo Mission captured images of Earth from space for the very first time. These iconic photos gave people around the world a fresh perspective on our home planet — more specifically, its vast and dazzling expanses of blue. It's perhaps unsurprising that science has subsequently established the key roles that the ocean and its marine organisms play in maintaining a planetary environment suitable for life.
While the Apollo astronauts were sending back pictures of our blue planet, a scientist at the Jet Propulsion Laboratory in California was searching for ways to detect life on other planets such as Mars. James Lovelock's investigations led him to conclude that the only way to explain the atmospheric composition of Earth was that life was manipulating it on a daily basis. In various publications, including his seminal 1979 book Gaia: A New Look at Life on Earth, Lovelock launched the Gaia hypothesis, which describes how the physical and living components of the natural environment, including humankind, interact to maintain conditions on Earth. During the same period, marine scientists including Lawrence Pomeroy, Farooq Azam and Hugh Ducklow were establishing a firm link between the major biogeochemical cycles in the oceans and marine food webs, particularly their microbial components. In the late 1980s and 1990s, large-scale research programs like the Joint Global Ocean Flux Study (JGOFS) explored ocean biogeochemistry and established the oceans' pivotal role in the Earth's carbon cycle.
Research efforts like these underscored the oceans' critical importance in regulating all the major nutrient cycles on Earth. It's now widely recognized that the ocean regulates the temperature of Earth, controls its weather, provides us with oxygen, food and building materials, and even recycles our waste.
The advent of deep-sea science
It seems remarkable that until fairly recently many scientists believed that life was absent in the deep sea. Dredging in the Aegean Sea in the 1840s, marine biologist Edward Forbes found that the abundance of animals declined precipitously with depth. By extrapolation he concluded that the ocean would be azoic (devoid of animal life) below 300 fathoms (~550m depth). Despite evidence to the contrary, scientists supported the azoic hypothesis, reasoning that conditions were so hostile in the deep ocean that life simply could not survive. Extreme pressure, the absence of light and the lack of food were viewed as forming an impenetrable barrier to the survival of deep-sea marine species.
But others were already proving this hypothesis wrong. As Edward Forbes published his results from the Aegean, Captain James Clark Ross and the famous naturalist John Dalton Hooker were exploring the Antarctic in the Royal Navy vessels HMS Terror and HMS Erebus. During this expedition, Ross and Hooker retrieved organisms from sounding leads at depths of up to 1.8km, including urchin spines and other fragments of various marine invertebrates, a number of bryozoans and corals. Ross remarked, "I have no doubt that from however great a depth we may be enabled to bring up the mud and stones of the bed of the ocean we shall find them teeming with animal life." This contention was supported by work of Norwegian marine biologists Michael Sars and George Ossian Sars who dredged hundreds of species from depths of 200 to 300 fathoms off the Norwegian coast.
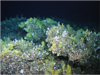
Further evidence came from natural scientists William Carpenter and Charles Wyville-Thomson, who mounted expeditions in 1868 and 1869 on the vessels HMS Lightening and HMS Porcupine to sample the deep ocean off the British Isles, Spain and the Mediterranean. The findings of these expeditions, which Wyville-Thomson published in his 1873 book The Depths of the Sea, confirmed the existence of animal life to depths of 650 fathoms — including all the marine invertebrate groups — and suggested that oceanic circulation exists in the deep sea.
This convinced the Royal Society of London and the Royal Navy to organize the circumnavigating voyage of HMS Challenger in the 1870s. In part, the expedition's purpose was to survey potential routes for submarine telegraph cables, and so the links between scientific exploration and human use of the deep sea were established in the very early days of oceanography. The Challenger expedition was a watershed for deep-ocean science, establishing the basic patterns of distribution of deep-sea animals, and that their main food source was the rain of organic material from surface waters.
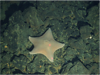
In the 1950s, the Danish Expedition Foundation's Galathea voyage established that life occurred at depths of more than 10km in the Philippines Trench. In 1960 marine explorers Auguste Picard and Don Walsh reached the bottom of the Challenger Deep in the Marianas Trench, at a depth estimated to be 10,916 meters--the deepest part of the ocean — where they observed flatfish from the porthole of their pressure sphere. This feat was not repeated until 2012 when James Cameron visited the bottom of the Challenger Deep in the submersible Deepsea Challenger.
Hype or hyper-diversity in the deep sea?
While working at Woods Hole Oceanographic Institution in the late 1960s, scientists Howard Sanders and Robert Hessler developed new types of deep-sea trawls called epibenthic sleds that featured extra- fine mesh in the nets. When the new trawls were tested, they recovered an astonishing diversity of species from the deep sea. It became apparent that the species richness of deep-sea communities actually increased with greater depth to a peak somewhere on the continental slope between 2,000 and 4,000 meters depth. Beyond these depths, diversity appeared to decrease (but not everywhere), or the pattern was unclear.
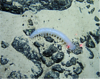
How to explain this amazing diversity in the deep sea? Initially, scientists credited the species richness to the stability of environmental conditions in the deep ocean, which would support extreme specialization of the animals and thus allow many species to coexist. This is known as the stability-time hypothesis. Some scientists considered that small-scale variations of the sediments of the deep ocean, including reworking of seabed by animals, was important in maintaining microhabitats for many species. In the late 1970s other scientists suggested that conditions in shallow waters allow competitive exclusion, where relatively few species dominate the ecosystem, whereas in deeper waters environmental factors associated with depth and a reduced food supply promote biological communities with more diversity.
Fred Grassle and Nancy Maciolek added substantially to our knowledge of deep-sea biodiversity when they published a study of the continental slope of the eastern coast of the USA in the early 1990s. Grassle and Maciolek based their study on quantitative samples of deep-sea sediments taken with box cores. These contraptions retrieve a neat cube-shaped chunk of the seabed and bring it to the surface enclosed in a steel box. Scientists then sieve the mud and count and identify the tiny animals living in the sediment.
In a heroic effort, Grassle and Maciolek analyzed 233 box cores, an equivalent of 21 square meters of the seabed, identifying 90,677 specimens and 798 species. They estimated that they found approximately 100 species per 100 km along the seabed they sampled. Extrapolations of this figure suggested that there may be 1 - 10 million macrofaunal species in the deep sea.
What's more, some scientists argued that Grassle and Maciolek's estimates represented only a small part of the species diversity in the ocean depths. Dr John Lambshead of London's Natural History Museum pointed out that Grassle and Maciolek had not examined the smallest animals in sediments — the meiofauna — made up of tiny nematode worms, copepods and other animals. These are at least an order of magnitude more diverse than the macrofauna, suggesting that as many as 100 million species may inhabit the deep ocean.
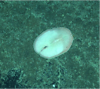
However, given that the latest approximation of the Earth's biodiversity is 10 million species in total, Lambshead's number appears to be an overestimate. Scientists have since realized that there are major problems with estimating the species richness of large areas of the deep sea based on local samples. Today we understand that species diversity in the deep ocean is high, but we still don't know how many species live in the sediments of the continental slope and abyssal plains. We also don't understand the patterns of their horizontal distribution or the reasons for the parabolic pattern of species diversity as it relates to depth. Evidence suggests, however, that the functioning of deep-sea ecosystems depends on a high diversity of animals — although exactly why remains open to conjecture.
The creation of deep-sea environments: "Drifters" and "Fixists"
In 1912, German scientist Alfred Wegener put forward his theory of continental drift to address many questions that engaged the geologists and biologists of his time. For example, why do the continents appear to fit together as though they had once been joined? Why are many of the large mountain ranges coastal? And, perhaps most intriguing, why do the rocks and fossil biotas (combined plant and animal life) on disconnected land masses appear to be so similar?
Wegener's theory provoked a major scientific controversy that raged for more than 50 years between "drifters" and "fixists." Critics of Wegener's — the "fixists" — pointed out that Wegener's proposed mechanism for drift was flawed.
In the search for an alternate mechanism to explain continental drift, British geologist Arthur Holmes suggested that radioactive elements in the Earth were generating heat and causing convection currents that made the Earth's mantle fluid. Holmes argued that the mantle would then rise up under the continents and split them apart, generating ocean basins and carrying the landmasses along on the horizontally-moving currents.
Following World War II, scientific expeditions employing deep-sea cameras, continuously recording echo-sounders, deep-seismic profilers and magnetometers lent support to the arguments of Holmes and his fellow "drifters." Scientists realized that the deep sea hosted a vast network of mid-ocean ridges located roughly in the center of the ocean basins. These ridges were characterized by fresh pillow lavas, sparse sediment cover, intense seismic activity and anomalously high heat flow. Scientists found geologically-synchronous magnetic reversals in the rocks of the ocean crust moving away from either side of the mid-ocean ridges. Added to this was the fact that nowhere could scientists find sediments older than the Cretaceous in age. Together, these findings suggested that new oceanic crust was being formed along the mid-ocean ridges, while old oceanic plates are forced underneath continental plates and destroyed along the ocean trenches. By the late 1960s, the bitter scientific debate between the "fixists" and the "drifters" was finally settled.
Life without the sun
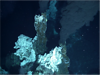
During the next decade, scientists investigating volcanic activity at mid-ocean ridges became interested in the associated phenomenon of hot springs in the deep sea. Anomalously high temperature readings over mid-ocean ridge axes led scientists to mount an expedition in 1977 to the 2.5 km-deep Galápagos Rift. From the submersible Alvin, the scientists observed plumes of warm water rising from within the pillow lavas on the seabed. Living amongst the pillows were dense communities of large vesicoyid clams, mussels, limpets and giant vestimentiferan tube worms (Siboglinidae). An abundance of bacteria around the Galápagos Rift site immediately suggested that these communities might be based on bacterial chemosynthesis, or chemolithotrophy, using chemical energy obtained by oxidizing hydrogen sulphide to drive carbon fixation. Subsequent investigation confirmed that the giant tube worms, clams and mussels actually hosted symbiotic sulphur-oxidizing bacteria in their tissues.
The discovery caused huge excitement in the scientific community. Here was life thriving in the deep sea, where primary production — the basis of the food web — was independent from the sun's energy. Furthermore, as scientists discovered additional vent communities and surveyed elsewhere in the mid-ocean ridge system, they found that environmental conditions were extreme, with high temperatures, acidic waters, hypoxia (lack of oxygen) and the presence of toxic chemicals the norm.
The implications of this were enormous and went well beyond the study of the ocean itself. First, it meant that life could exist elsewhere in our solar system in environments previously thought too extreme. Second, it widened the potential area for habitable planets around suns elsewhere in the universe. For example, the discovery in 2000 of the Lost City alkaline hydrothermal vents presented an environment that some scientists suggest is analogous to the conditions in which life evolved on Earth.
Subsequently, chemosynthesis has been discovered in many places in the ocean, including deep-sea hydrocarbon seeps, in large falls of organic matter such as whale carcasses, and from shallow-water sediments associated with, for example, seagrass beds.
Drawing down the oceans' natural capital
Over the past two decades, we've developed a much deeper understanding of the relationship between humankind and the natural world, including the Earth's oceans. In 1997 Robert Costanza and his colleagues published a paper in Nature that estimated the economic value of the goods and services provided by global ecosystems. Costanza and his colleagues argued that the living resources of Earth could be viewed as a form of natural capital with a value averaging $33 trillion per annum, upon which the entire human economy depended. These goods and services were later grouped into supporting (e.g. primary production), provisioning (e.g. food), regulating (climate regulation) and cultural (e.g. education) services.
While this knowledge may have been intuitive for many people, Costanza's recasting of the environment in economic terms forced policymakers, industry leaders and others to recognize the importance of long-term environmental sustainability. With the support of international agencies such as the World Bank, many countries are now implementing natural capital accounting procedures through legislation. The purpose of this is to help monitor and regulate the use and degradation of the environment and to ensure that the critical ecosystem goods and services underpinning economic activity and human well-being are not undermined.
Although it seems like a modern preoccupation, sustainability is actually a centuries-old challenge, particularly as it relates to marine environments. For example, there is evidence that aboriginal fisheries in ancient times may have overexploited marine species. Certainly by medieval times in Europe, a thriving market for fish, coupled with other developments like changing agricultural practices, forced species such as salmon and sturgeon into decline.
The Industrial Revolution led to an increase in hunting fish, seals and whales, thanks to the development of steam- and then oil-powered fishing vessels that employed increasingly sophisticated means of catching animals. Pelagic whaling began in the early 20th century; the development of explosive harpoons, the ability to process whales at sea, and the strong demand for margarine made from whale oil all contributed to dramatic rises in catches. Despite the initiation of the International Whaling Commission in 1946, a serial depletion of whale populations took place from the largest, most valuable species (e.g. blue whale) through to the smallest species (minke whale). The failure to regulate catches of whales led to the establishment of a near-moratorium on whaling in 1986.
Over the same post-war period, fishing fleets underwent a major expansion and deployed increasingly powerful fishing vessels. Improved technologies for navigating, finding fish and catching them led to increasing pressure on fish stocks and the marine ecosystems in which they lived. In 1998, after analyzing catch statistics from the United Nations Food and Agricultural Organisation (FAO), Daniel Pauly and his colleagues from the University of British Columbia identified a global shift in fish catches from long-lived, high trophic level predators to short-lived, low trophic level invertebrates and plankton-eating fish. This was the first evidence that fishing was having a global impact on marine ecosystems, causing major changes in the structure of ocean food webs. Aside from the economic impacts of "fishing down the food web," evidence was accumulating that it also affected the vulnerability and/or resilience of marine ecosystems to shocks such as invasions by alien species and climate-change effects such as mass coral bleaching.
Further evidence came in 2003 from a study by Ransom Myers and Boris Worm. Myers and Worm documented a significant decline over time in the stocks of certain large, predatory fish after analyzing information from research trawl surveys and the catches of the Japanese long-line fleet. Other studies over the same time period suggested that sharks, seabirds and turtles were suffering large-scale declines as they became by-catch in many industrial fisheries. Scientists also asserted that some fishing technologies, such as bottom trawling, were extremely damaging to seabed communities — deep-sea ecosystems in particular — by documenting the devastation of cold-water coral communities.

These studies sparked a bitter war of words between marine ecologists, fishing industry executives and fisheries biologists. While it has now been demonstrated that fish stocks can recover if levels of exploitation by fisheries are reduced through management measures, it's clear that in many parts of the world's oceans this is not happening. Overall, global yields from marine capture fisheries are in a downward trajectory. By-catch of some marine predators, such as albatrosses, still poses a threat of extinction. Habitat destruction resulting from fishing is continuing.
In addition to overfishing, other human activities are damaging marine ecosystems. During the 1960s and 1970s, several major accidents with oil tankers and oil installations resulted in serious oil spills. While oil pollution is still a significant problem, as illustrated by the Deepwater Horizon disaster in the Gulf of Mexico in 2010, other less-visible sources of pollution are causing large-scale degradation of the ocean.
Persistent organic pollutants and heavy metals such as mercury are being recognized as major health issues for marine animals (especially high trophic level predators, such as killer whales and tuna) and also for humans. The oceans are becoming the dumping ground for a wide range of chemicals from our personal care products and pharmaceuticals, as well as those that leach out of all manner of plastics that are floating in our seas. Agrochemicals are pouring into the oceans through rivers; in some cases these artificially fertilize coastal waters, generating blooms of algae which are broken down by bacteria, thus stripping the water of oxygen and creating dead zones.
Our release of greenhouse gases into the atmosphere, particularly carbon dioxide (CO2), is leading to a profound disturbance in ocean temperatures and ocean chemistry. Since the late 1970s, mass coral bleaching from ocean warming has killed large areas of tropical coral reefs. Marine animals are changing their distribution and the timing of their lifecycles, sometimes with catastrophic effects across the wider ecosystem. Such effects are often propagated from lower levels of food webs up through to predators such as fish and seabirds: witness recent declines in spectacled sea duck populations in the Arctic and the decline of cod populations in the North Sea. The oceans are becoming more acidic, which affects the growth rates of animals with calcium carbonate shells or skeletons and has other negative impacts on animal physiology. Many of these different stresses on marine species interact in a form of "negative synergy", inducing more severe effects than if they had presented in isolation. At the ecosystem level these stresses reduce the resilience of marine ecosystems to "shocks" arising from large-scale effects, such as anomalous warming events associated with climate change.
Ocean future
The TEDTalks in The Deep Ocean illuminate many current topics in marine science and oceanic exploration. These include the call for better conservation management in the face of unprecedented threats to marine ecosystems, the discovery and application of as-yet-untapped natural resources from the ocean depths, and the quest for improved technologies to support both of these endeavors. As Sylvia Earle eloquently reminds us in her 2009 TEDTalk, the oceans are critically important to maintaining the planet in a condition that is habitable, and better cooperative, international management of marine ecosystems is essential. However, as other TED speakers like Robert Ballard and Craig Venter argue, the oceans should also interest us because they contain vast untapped resources: unexploited mineral resources as well as genes, proteins and other biomolecules of marine life, which may furnish the medicines and industrial materials of the future.
Smart management of these natural resources requires knowledge, as do our efforts to ensure the oceans' ongoing species richness and their critical function in maintaining the Earth system. In their TEDTalks, explorers and scientists Edith Widder, Mike deGruy and Craig Venter share some of the amazing physical and biological features of ocean habitats and describe how new technologies allow more careful study and exploitation of deep-sea environments.
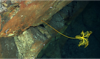
Despite these advances, there are still enormous gaps in our knowledge. In a TEDTalk he gave in 2008, Robert Ballard noted that many parts of the ocean remain entirely unexplored and he advocated for increased resources for organizations like NOAA. As many of the TED speakers in The Deep Ocean argue, marine science is more important than ever because the oceans are under serious threat from a range of human impacts including global-scale climate change.
However, these speakers also offer a message of hope, underscoring that there is still time to alter the current trajectory of degradation. Scientists including TED speaker John Delaney present a vision for the future where ecosystem-based management, coupled with the advent of new technologies that allow us to monitor ocean health in real time, provide us with tools to heal marine ecosystems. This may allow us to restore their capacity to provide goods and services for humankind over the long term. Measures such as marine-protected areas can maintain the oceans' important biogeochemical functions, but will also conserve the remarkable and beautiful marine ecosystems that have culturally enriched the human experience for millennia.
We'll begin our journey into The Deep Ocean with legendary explorer and oceanographer Sylvia Earle, who shares disturbing data about the decline of marine ecosystems and proposes one method to protect what she calls "the blue heart of the planet."
Relevant talks